ILTER Nitrogen Initiative Training Course 2016

Changing nitrogen cycles in ecosystems across the globe:
Training the next generation of ILTER scientists
Hideaki Shibata1), Peter M. Groffman2), William H. McDowell3), Pedro Pinho4), Karibu Fukuzawa1), Makoto Kobayashi1), Yoshitaka Uchida5), Keisuke Koba6), Toshiya Yoshida1), Yoshitaka Ohishi7), Yiching Lin8), Chiling Chen9), I-Ling Lai10), Chiao-Ping Wang11), Wei-Chun Chao12), Chung-Te Chang13), Jinanwu Tang14), Pamela H. Templer15), Ika Djukic16), Kazuo Isobe17), Hideyuki Doi18), Ayato Kohzu19), Yoshiharu Fujita20), Tsutom Hiura1), Nobuhito Ohte21), Adriana C. Flores-Díaz22), Andreea Csolti23), Ei Thandar Bol24), I-Jou Hsiao8), Ikabongo Mukumbuta5), Ilann Bourgeois25), Jinsen Zheng24), Joseph C. Morina26), Jun-Wei Lu8), Lucy Ann Rose27), Nanae Hosokawa28), Rahat Sharif29), Rebecca E. Hewitt30), Silvia Rafaela Machado Lins31), Takahiro Inoue28), Undrakh-Od Baatar32), Wei Zhou33), Wei-yu Shi34), Yoshio N. Palma35), Ming-Chien Su36)
Affiliations:1) Field Science Center for Northern Biosphere, Hokkaido University, Japan, 2) City University of New York, Advanced Science Research Center and Brooklyn, College Department of Earth and Environmental Sciences, USA 3) University of New Hampshire, USA, 4) University of Lisbon, Portugal, 5) Graduate School of Agriculture, Hokkaido University, Japan, 6) Center for Ecological Research, Kyoto University, Japan, 7) Center for Arts and Sciences, Fukui Prefectural University, Japan, 8) Tunghai University, Taiwan, 9) Taiwan Agricultural Research Institute, Taiwan, 10) National Pingtung University of Science and Technology, Taiwan, 11) Taiwan Forestry Research Institute, Taiwan, 12) National Chiayi University, Taiwan, 13) National Taiwan University, Taiwan, 14) The Ecosystem Center, Marine Biological Laboratory, USA, 15) Boston University, USA, 16) Environment Agency, Austria, 17) The University of Tokyo, Japan, 18) University of Hyogo, Japan, 19) National Institute for Environmental Studies, Japan, 20) Center for Open Education, Hokkaido University, Japan, 21) Graduate School of Informatics, Kyoto University, Japan, 22) Institute for Research on Ecosystems and Sustainability UNAM, Mexico, 23) University of Bucharest, Romania, 24) Graduate School of Agriculture, Kyoto University, Japan, 25) Université Grenoble-Alpes, France, 26)Virginia Commonwealth University, USA, 27) University of Minnesota Twin Cities, USA, 28) Graduate School of Environmental Science, Hokkaido University, Japan, 29) University of Maryland - College Park, USA, 30) Northern Arizona University, USA, 31) University of Sao Paulo, Brazil, 32) University of Vienna, Austria 33) Institute of Soil Sciences, Chinese Academy of Sciences, China, 34) Institute of Earth Environment, Chinese Academy of Sciences, China, 35) Tokyo Institute of Technology, Japan, 36) National Dong-Hua University, Taiwan
Abstract: Nitrogen is an essential nutrient for all biota, but in excess behaves as a pollutant in the environment. The doubling of total reactive nitrogen (nitrogen not in the form of N2 gas) pools globally has resulted in many undesirable changes in aquatic and terrestrial ecosystems. The International Long-Term Ecological Research (ILTER) network, a global site and researcher network focused on long-term, site-based ecosystem and socio-ecological research, organized a nine-day international training course in Hokkaido, Japan to expose young researchers to state-of-the-art analysis of nitrogen cycling in ecosystems with a focus on key ecosystem processes and implications for environmental pollution. This training course was designed by the ILTER Nitrogen Initiative and yielded several important insights for both science and education. First is the realization that we are on the threshold of a new era of global scale science related to nitrogen from various perspectives. Our experience suggests that the ILTER nitrogen project is well poised to produce insights that are relevant to coupled regional and global scale dynamics and provides a strong context and motivation for a multi-national training course. Second, the ILTER nitrogen training course in Japan showed that a considerable amount of site-specific and synthetic science can be accomplished in such a course. Our students carried out two exercises, 1) a data synthesis exercise where they developed questions and then searched for and analyzed existing data available on ILTER websites and 2) field measurements including N2O emission, soil N cycles, and earthworm populations, at a well-studied research site in northern Japan. Finally, the ILTER nitrogen training course in Japan highlights that in-person, on site collaborative interactions between students and faculty is still the “gold standard” for high impact, high level education. Allowing students to interact with active scholars in lecture, laboratory, and field settings, and having faculty available as students develop group projects, produces clearly exceptional educational experiences. The training course will help to create a cadre of scientists better able to address global scale questions and organize across political boundaries and jurisdictions.
Keywords: Biogeochemistry, Denitrification, Earthworm, N2O emission, Mosses, Soil N mineralization, Stable isotope
1. Nitrogen: An emergent issue across the globe
Changes in the global nitrogen cycle have been among the most obvious and consequential changes in the global environment over the last half a century. During this time the global supply of reactive nitrogen has doubled (Galloway et al. 2008). Although the vast bulk of nitrogen on earth is in atmospheric pools of N2 gas that are relatively inaccessible to plants and animals, nitrogen in the forms of ammonium, nitrate, and organic nitrogen is central to the productivity of the globe. This so-called reactive nitrogen is essential to life, and often limits crop production or the productivity of algae in coastal waters. The primary drivers increasing the pool of reactive nitrogen have been fertilizer production and application to meet agricultural needs, and atmospheric deposition of nitrogen that has been produced burning of fossil fuels.
The doubling of total reactive nitrogen pools globally has resulted in many undesirable changes in aquatic and terrestrial ecosystems (Shibata et al. 2015). These include production of anoxic “dead zones” in many coastal waters such as the Gulf of Mexico, which receives large amounts of reactive nitrogen and other nutrients from upstream agricultural production in the heartland of the United States. These dead zones can be the result of naturally occurring processes, but the extent, severity, and impact of dead zones on coastal ecosystems has increased over the past decades. Dead zones are now found in most coastal areas, with a strong correlation between intense human land use, nutrient levels in rivers, and the presence of dead zones on the coast (Diaz and Rosenberg 2008).
Even though small additions of reactive nitrogen can enhance plant productivity, its application to terrestrial systems via atmospheric deposition can alter natural forest communities in many ways. Excess N can cause large changes in overall carbon and nutrient cycling (e.g. Cusack et al. 2011; Frey et al. 2014) and can even result in wholesale changes in forest composition due to tree death (Aber et al. 1998). More subtle effects of increased atmospheric deposition of N are not as well documented, but include changes in the community composition of sensitive species such as lichens (Pinho et al. 2011).
Another undesirable outcome of the global increase in reactive nitrogen has been the increased production of nitrous oxide (N2O) during crop production and animal husbandry (e.g. Robertson et al. 2014). Nitrous oxide is a globally important greenhouse gas, with much larger greenhouse effects per molecule than carbon dioxide. Because its production is associated with various pathways in the nitrogen cycle, and is widely variable in space and time, quantifying the global rate of N2O production is a central and ongoing challenge for biogeochemists. Production of N2O is sensitive to environmental conditions (e.g., extent of reducing conditions, pH, and water and carbon availability), as well as the overall amounts of reactive nitrogen cycling through an ecosystem. Efforts are being made to reduce emissions of N2O through changes in agricultural practices (Robertson et al. 2014), but much work remains before N2O production can be effectively managed and reduced at the landscape scale.
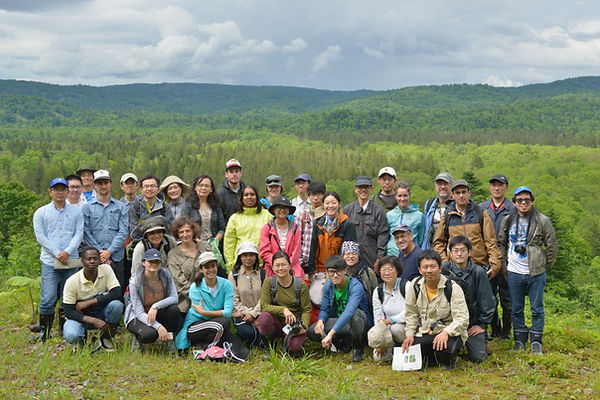
Photo 1. Group photo taken during the ILTER-N training course at the Uryu Experimental Forest (JaLTER core-site) of Hokkaido University, Japan, June 21st, 2016.
2. ILTER and training course
The International Long-Term Ecological Research (ILTER) network is a global site and researcher network focused on long-term, site-based ecosystem and socio-ecological research (https://www.ilternet.edu/). The ILTER was established in 1993 and currently comprises over 700 sites across the globe. The ILTER-Nitrogen Initiative was founded at the ILTER annual meeting in Sapporo, Japan in 2011 and is focused on understanding altered nitrogen biogeochemistry and its impact in different environments and socio-ecological settings at an international scale. The ILTER-Nitrogen Initiative is driven by an overarching question; “what are the ecosystem responses to reactive nitrogen changes across global ecosystems?” with three focal topics (i) the use of lichens for bio-monitoring of nitrogen pollution, (ii) global analysis of the factors driving N2O emission from soils, and (iii) understanding the long-term legacy impact (i.e. previous environmental changes and various human perturbations) on current nitrogen cycles and budgets locally, regionally, and globally.
The ILTER Nitrogen training course was designed to expose young researchers to state-of-the-art approaches to analysis of nitrogen cycling in ecosystems with a focus on key ecosystem processes and implications for environmental pollution. The course took place at Hokkaido University in Sapporo, Japan and the Uryu Experimental Forest of Hokkaido University in Japan (a core-site of Japan LTER network) from June 16-24th, 2016. Nineteen participants from eight ILTER network countries (Japan, Taiwan, USA, Mexico, France, Brazil, Austria and China) attended this course. Twenty four academic staff from Japan, Taiwan, USA, Portugal and Austria gave a series of indoor lectures and field demonstrations on cutting-edge findings, research protocols, and meta-analysis tools related to long-term nitrogen cycles in ecosystems. The students worked in small groups and carried out two projects. First, they searched for, downloaded and analyzed existing ILTER data and they then carried out intensive data collection in forest research sites at the Uryu experimental forest. Both efforts were driven by specific research questions and hypotheses. These short, but intensive group effort projects produced a range of results and insights as described below.
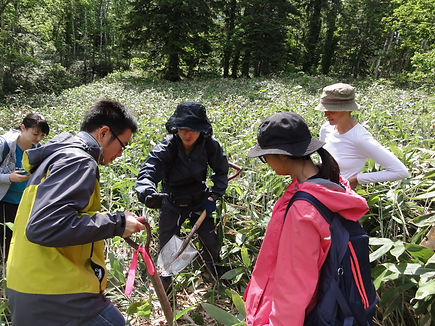
Photo 2. Group work for field data collection.
3. Snapshot of the preliminary findings and implications from data collection at Uryu Experimental Forest
3.1 Earthworm and N2O emission
Nitrous oxide is an important and often overlooked greenhouse gas, mostly emitted from soil. Previous studies have shown a positive correlation between abundance of earthworms in agricultural soils and N2O emissions (Lubbers et al. 2013). However, little work has been done in forests, especially under natural forest succession after various disturbances. Here, we hypothesized that differences in earthworm abundance are linked to N2O flux at all stages of natural succession in forests. This hypothesis was tested across a successional gradient in the Uryu Experimental forest including Sasa dwarf bamboo forest (dominated by Sasa senanensis) developed under the large tree-canopy gap, primary mixed forest (dominated by Picea glehnii, Abies sachalinensis and some deciduous species) and secondary forest (dominated by Betula platyphylla) with the primary disturbance either clear-cutting and/or natural disturbances such as windthrow associated with typhoons. In the three forest types, three replicate trace gas flux chambers were installed and three replicate soil samples were taken for earthworm analysis (total 9 samples). Gas samples were taken at two time periods, an initial sample immediately after the chamber was placed above the soil, and a second one hour later. Gas samples were analyzed using a Los Gatos Isotopic N2O Analyzer (Model No. 914-0027). Soil bulk density, moisture, pH and temperature were also determined at the same site locations. Earthworms were hand collected by digging a 0.25 m2 area to 30 cm depth.
In univariate analysis there was a tendency for N2O to increase with soil temperature and earthworm population abundance, and to decrease with higher soil pH and percent bamboo cover. The negative relationship between N2O emissions and bamboo cover could be caused by differences in soil pH that in turn would influence earthworm abundance and activity (Figure 1). In fact, after accounting for the influence of “site” using a generalized linear mixed model, a significant effect of pH on earthworm abundance was observed. Warmer temperatures may increase earthworm activity, including digestion, which could lead to more decomposition and release of N2O. The next step of this study is to increase the number of replicates and test under control experiments the direct link between N2O emissions and earthworm population size.
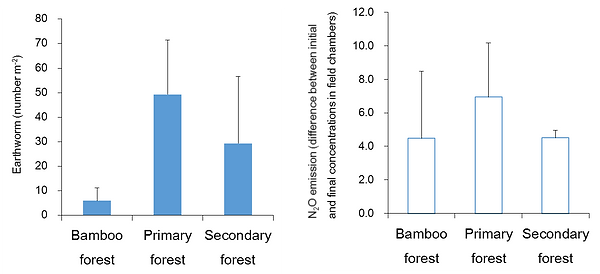
Figure 1. Number of earthworms (left panel) and N2O emission (right panel; change in N2O between initial and final concentrations in field chambers) in bamboo forest (Sasa spp.), primary forest (cool-temperate mixed forest) and secondary forest (Betula spp.) sites in the M3 experimental watershed of the Uryu Experimental Forest. Bars are mean ± standard deviation.
3.2 Effect of N addition on N2O emission
Nitrous oxide emission from soil is driven by multiple environmental factors, such as temperature, moisture, soil pH, microbial composition, as well as substrate and nutrient availability. The significance of these drivers often relies on the site-specific conditions in each ecosystem. The emergent question for this topic during the course was, “how does nitrous oxide production vary in peat soils when nitrate or ammonium availability is high?” We hypothesized that nitrate additions increase nitrous oxide production relative to control and ammonium additions. Because of the reduced (i.e. low oxygen availability) soil conditions of our sites, ammonium was not expected to increase nitrous oxide production via nitrification. We utilized a soil slurry technique to investigate how nitrous oxide production varied across treatments. Soils were collected from 40-60 cm below the soil surface from a peatland, roots were removed (10 minutes picking of roots larger than hair diameter), and remaining soils were homogenized and placed in laboratory bottles. Soils were treated with solutions containing high (25 mg N/L) concentrations of nitrate or ammonium or deionized water (control). Gas samples were collected from the headspace from each bottle immediately and 60 minutes of incubation, and then analyzed for N2O concentration using the Los Gatos Isotopic N2O Analyzer.

Photo 2. Group work for lab analysis
Control samples showed a negative rate of nitrous oxide flux (-2.6 ng N2O-N g dry soil-1 hr -1), suggesting consumption over the 60 minute incubation period (Figure 2). In contrast, samples treated with nitrate showed a positive rate of N2O flux over this time period (5.4 ng N2O-N g dry soil-1 hr -1). The ammonium-treated samples had a mean low positive rate (0.09 ng N2O-N g dry soil-1 hr -1), although deviation around the mean suggests both consumption and production of N2O. These results suggest that the majority of N2O production from peat soils could be attributed to anaerobic denitrification rather than aerobic nitrification. It is also implied that there is significant potential for nitrous consumption in these soils.

Figure 2. Rates of nitrous oxide flux from soil slurries collected at the Swamp forest dominated by Picea glehnii in Dorokawa watershed. The “NH4+” and “NO3-” represents the experimental addition of the ammonium nitrogen and nitrate nitrogen, respectively. The values and errors indicate mean and +1SD (N = 3).
3.3 Contribution of nitrification and denitrification to N2O emissions in upland moist and riparian wetland soils
It is well known that nitrification and denitrification both contribute to N2O emissions from soil, while the quantitative separation of those contributions is not well understood. We amended upland and riparian wetland soils (in a 1st order headwater catchment) with 5 g NH4+-N kg-1 and 5 g NO3--N kg-1 to characterize the relative contributions of nitrification and denitrification to soil N2O emissions. The goals of this project were to compare N2O emissions between wetland and upland forest sites, examine responses of N2O emissions to NH4+ and NO3- additions, and utilize natural abundance stable isotopes to partition nitrification vs denitrification as sources of N2O. Following a 1-hour incubation period, N2O emissions were generally higher in the wetland soil than the upland soil and the fraction of added N that was emitted as N2O was higher in the wetland soil compared to the upland soil. In the wetland soil, higher N2O concentrations generally occurred following amendment with NO3--N than with NH4+-N while in the upland soils, N2O concentrations were higher following the addition of NH4+-N. δ15N-N2O values decreased with increasing N2O concentration and were lower in the wetland soil than in the upland soil. The lowest δ15N-N2O values in the wetland soil occurred following NO3--N amendment. Patterns in isotopic substitution (“site preference”) suggested that denitrification was the dominant N2O source in wetland soils and that the pathway of N2O formation (nitrification vs. denitrification) was not strongly affected by the form of N amendment. Site preference values in the upland soils suggest greater contributions of nitrification to N2O emissions than in the wetland soils, with no clear patterns related to the form of N amendment. Across both ecosystem types, the increase in δ15N, δ18O, and absolute site preference values with decreasing N2O concentrations suggest that N2O reduction to N2 may be an important process in these soils. While the Los Gatos laser instrument rapidly provided interesting data, the accuracy of the stable isotope values for N2O were relatively more variable when compared to the previously reported values using isotope ratio mass spectrometry techniques. Although we were able to observe the “trend” of the isotopic values of N2O using the laser, further clarification of the concentration/moisture dependencies of the raw values obtained from the laser is critically important.

Figure. 3. N2O emission (increased concentration during the laboratory incubation) in riparian (Wetland) and upland (Upland) soils treated with either NH4+ or NO3- from the M3 watershed of the Uryu Experimental Forest. The point and box indicate median and ±25% quantile (N = 3).
3.4 The influence of mosses on N2O and soil mineral N
In northern forests, mosses are important to the nitrogen economy, especially inputs through fixation. Recently there is interest not only regarding the fixation, but also the emission of N2O from mosses. Our objectives were to i) compare the effects of presence/absence of mosses on N2O production and soil mineral N; and ii) compare the effect of moss functional types on N2O production and soil mineral N. We made observations of soil chemistry, moisture, and N2O fluxes from patches of peat forming mosses (dominant species Sphagnum fuscum) and feather mosses (dominant species Pleurozium schreberi). We compared these measurements to patches of bare ground at the edge of a bamboo (Sasa spp.) understory to assess the contribution of two moss functional types to soil mineral N and the production of N2O. We coupled field observations with laboratory measurements of N2O fluxes from incubated samples of sphagnum, feather moss, and bare ground at 7 °C warmer than field conditions for 17 hours to determine whether the flux potential differs under common conditions.

Figure 4. N2O emission from the Sphagnum, Feather moss, and bare ground in Dorokawa swamp forest when incubated for 17 hours at 7oC above ambient temperatures.
We found no statistically significant differences in N2O flux from different cover types, although there was a consistent tendency for feather mosses to emit N2O, while Sphagnum and bare ground did not (Figure 4). Sphagnum mosses emitted N2O at one site, but consumed it another site. The emissions observed during our incubation trial may be constrained by site conditions in the field. For example, in our incubation experiment, the mosses were removed from the soil surface and this disturbance effect could change their capacity in produce and/or consume N2O. We also incubated the mosses overnight in the dark therefore any link to photosynthesis that might influence gas emission patterns was eliminated. Future research should assess the distribution of common moss functional types along environmental gradients to determine the relative importance of biotic vs abiotic controls on N2O emissions in northern forests.
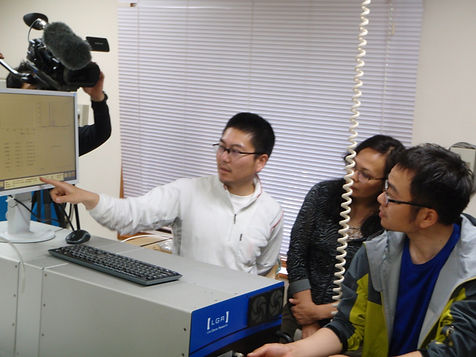
Photo 3. Group work for lab analysis using the Los-Gatos Isotopic N2O Analyzer to measure N2O concentration, stable isotope composition and isotope site preference.
4. Lessons and conclusion
Our experience with the ILTER nitrogen training course in Japan yielded several important insights for both science and education. First is the realization that we are on the threshold of a new era of global scale science related to nitrogen. Nitrogen is well recognized as a global scale problem, yet variation in the nature and extent of nitrogen pollution around the globe is extreme. While some areas (e.g., China, India, Africa) are on a rapid trajectory of increasing fertilizer inputs and atmospheric deposition, others (Europe, North America, Japan) are experiencing marked decreases in deposition and stabilization in rates of fertilizer use. There are great intellectual and practical challenges to global scale science. Intellectually, understanding dynamics at this scale requires multi- and trans-disciplinary analysis across atmospheric science, hydrology, biogeochemistry, microbial and plant ecology, and social science. Practically, developing funding for projects across international boundaries is complicated by national interests and limitations. Our experience suggests that the ILTER nitrogen project is well poised to continue to produce insights that are relevant to coupled regional and global scale dynamics and provides a strong context and motivation for a multi-national training course. More fundamentally, these training courses will help to create a cadre of scientists who have the knowledge to address global scale questions and organize across political boundaries and jurisdictions.
The second conclusion from our experience with the ILTER nitrogen training course in Japan is that a considerable amount of site-specific and synthetic science can be accomplished in such an intensive, short-term course. Our students carried out two exercises, 1) a data synthesis exercise where they developed questions and then searched for and analyzed existing data available on the internet and 2) field measurements at a well-studied research site in northern Japan. In addition to providing diverse hands-on experiences for the students, these exercises produced surprisingly novel and important scientific insights. As described in the “preliminary findings” section above, students were able to test novel ideas on the regulation of nitrogen dynamics at multiple scales utilizing datasets from sites around the world from which and they were able to produce new data on nitrogen cycle process rates using state-of-the-art methodology. These successes were clearly facilitated by careful planning by the host institution (Hokkaido University) and they highlight the potential for students to produce genuine scientific advances in the proper setting.
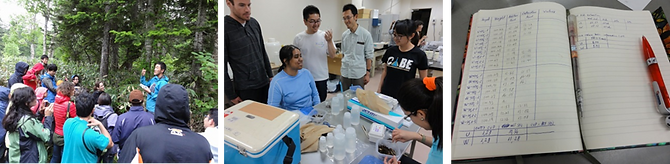
Photo 4. On-site collaborative interactions between students and faculty is still the “gold standard” for high impact, high level education.
Finally, our experience with the ILTER nitrogen training course in Japan highlights that in-person, on site collaborative interactions between students and faculty is still the “gold standard” for high impact, high level education. Allowing students to interact with active scholars in lecture, laboratory, and field settings, and having faculty available as students develop group projects, produces clearly exceptional educational experiences. While this is not a particularly surprising result, it is significant as we consider increasingly impersonal approaches to education, e.g., Massive Online Open Courses (MOOCs). It is important to keep this in mind as we address challenging, critically important topics such as global nitrogen pollution.
Acknowledgements: We thank ILTER, JaLTER (Japan LTER) and TERN (Taiwan Ecosystem Research) for co-organizing this training program. We also acknowledge funding support from the Environmental Research and Technology Development Fund (S-15) of the Ministry of the Environment, Japan; The U.S. National Science Foundation (Grant DEB-1642303); the Joint Usage / Research Grant of Center for Ecological Research (2016jurc-cer015), Kyoto University, Japan; the Joint Usage / Education grant of Filed Science Center for Northern Biosphere, Hokkaido University, “Field Studies of Forest Environment and Ecosystem Conservation (2012-2016)”; the Society of Subtropical Ecology; Ministry of Education, Taiwan; Tunghai University, Taiwan; International Long-Term Ecological Research Network (ILTER); FCT (SFRH/BPD/75425/2010), and Nitro, Portugal (H2020-TWINN 692331). We also thank the technical and administrative staff of Uryu Experimental Forest (a JaLTER core-site) of Hokkaido University for their helpful support and logistic arrangements for the training course. We thank Drs. Teng-Chiu Lin (Taiwan), Anna Avila Castells (Spain) and Tomoaki Morishita (Japan) for providing valuable research data for the group work at the course.
References:
-
Aber, J., W. McDowell, K. Nadelhoffer, A. Magill, G. Berntson, M. Kamakea, S. McNulty, W. Currie, et al. 1998. Nitrogen saturation in temperate forest ecosystems: Hypotheses revisited. BioScience 48: 921–934.
-
Cusack, D. F., W. L. Silver, and W. H. McDowell. 2011. Effects of chronic nitrogen additions on above- and belowground carbon dynamics in two tropical forests. Biogeochemistry. DOI 10.1007/s10533-010-9496-4.
-
Diaz, R. J. and R. Rosenberg (2008). "Spreading dead zones and consequences for marine ecosystems." Science 321(5891): 926-929.
-
Frey, S.D., S. Ollinger, K. Nadelhoffer, R. Bowden, E. Brzostek, A. Burton, B. A. Caldwell, S. Crow, C. L. Goodale, A. S. Grandy, A. Finzi, M. G. Kramer, K. Lajtha, J. LeMoine, M. Martin, W. H. McDowell, R. Minocha, J. J. Sadowsky, P. H. Templer, and K. Wickings. 2014. Chronic nitrogen additions suppress decomposition and sequester soil carbon in temperate forests. Biogeochemistry 121:305-316.
-
Galloway, J.N., A.R. Townsend, J.W. Erisman, M. Bekunda, Z. Cai, J.R. Freney, L.A. Martinelli, S.P. Seitzinger, et al. (2008) Transformation of the Nitrogen Cycle: Recent Trends, Questions, and Potential Solutions. Science 320: 889–892.
-
Lubbers, I.M., E. López Gonzáleza, E.W.J. Hummelinkc, J.W. Van Groenigena. 2013. Earthworms can increase nitrous oxide emissions from managed grassland: A field study. Agriculture, Ecosystems and Environment 174: 40–48.
-
Pinho, P., T. Dias, C. Cruz, Y.S. Tang, M.A. Sutton, M.-A. Martins-Loucáo, C. Máguas, and C. Branquinho. 2011. Using lichen functional-diversity to assess the effects of atmospheric ammonia in Mediterranean woodlands. Journal of Applied Ecology 48: 1107–1116.
-
Robertson GP, Gross KL, Hamilton SK, Landis DA, Schmidt TM, Snapp SS, and SSwinton SM (2014) Farming for Ecosystem Services: An Ecological Approach to Production Agriculture. BioScience 64(5): 404-415.
-
Shibata H, Branquinho C, McDowell WH, Mitchell MJ, Monteith DT, Tang J, Arvola L, Cruz C, Cusack D, Halada L, Kopáček J, Máguas C, Sajidu S, Schubert H, Tokuchi N, Záhora J (2015) Consequence of altered nitrogen cycles in the coupled human and ecological system under changing climate: The need for long-term and site-based research. AMBIO 44(3):178-193 DOI 10.1007/s13280-014-0545-4